The aurora
is a glow observed in the night sky, usually in the polar zone. It
is also known as "northern lights" or "aurora borealis," which is
Latin for "northern dawn" since in Europe especially, it often
appears as a reddish glow on the northern horizon as if the sun were
rising from an unusual direction. The aurora borealis most often
occurs from September to October and March to April. Its southern
counterpart "aurora australis," has similar properties, so scientists prefer "polar
aurora" (or "aurora polaris").
Auroral
mechanism
The aurora is now known to be caused
by electrons of typical energy of 1-15 keV, i.e. the energy obtained
by the electrons passing through a voltage difference of 1000-15,000
volts. The light is produced when they collide with atoms of the
upper atmosphere, typically at altitudes of 80-150 km. It tends to
be dominated by emissions of atomic oxygen--the greenish line at
557.7 nm and (especially with electrons of lower energy and higher
altitude) the dark-red line at 630.0 nm. Both these represent
"forbidden" transitions of atomic oxygen from energy levels which
(in absence of collisions) persist for a long time, accounting for
the slow brightening and fading (0.5-1 sec) of auroral rays. Many
other lines can also be observed, especially those of molecular
nitrogen, and these vary much faster, revealing the true dynamic
nature of the aurora.
Auroras can also be observed in the ultra-violet (UV) light, a very
good way of observing it from space (but not from ground--the
atmosphere absorbs UV). The "Polar" spacecraft even observed it in
X-rays. The image is very rough, but precipitation of high-energy
electrons can be identified.
return to top
Auroral forms and magnetism
Typically the aurora appears either
as a diffuse glow or as "curtains" that approximately extend in the
east-west direction. At some times, they form "quiet arcs," at
others ("active aurora") they evolve and change constantly. Each
curtain consists of many parallel rays, each lined up with the local
direction of the magnetic field lines, suggesting that aurora is
shaped by the Earth's magnetic field, Indeed, satellites show
auroral electrons to be guided by magnetic field lines, spiraling
around them while moving earthwards.
The curtains often show folds called "striations." When the field
line guiding a bright auroral patch leads to a point directly above
the observer, the aurora may appear as a "corona" of diverging rays,
an effect of perspective.
In 1741 Hiorter and Celsius first noticed other evidence for
magnetic control, namely, large magnetic fluctuations occurred
whenever the aurora was observed overhead. This indicates (it was
later realized) that large electric currents were associated with
the aurora, flowing in the region where auroral light originated.
Kristian Birkeland (1903) deduced that the currents flowed in the
east-west directions along the auroral arc, and such currents,
flowing from the dayside towards (approximately) midnight were later
named "auroral electro-jets." (see also Birkeland currents).
Still another evidence for a magnetic connection are the statistics
of auroral observations. Elias Loomis (1860) and later in more
detail Hermann Fritz (1881) established that aurora appeared mainly
in the "auroral zone," a ring-shaped region of approx. radius 2500
km around the magnetic pole of the Earth, not its geographic one. It
was hardly ever seen near that pole itself. The instantaneous
distribution of aurora ("auroral oval," Yasha Feldstein 1963) is
slightly different, centered about 3-5 degrees night-ward of the
magnetic pole, so that auroral arcs reach furthest equator-ward
around midnight.
return to top
Frequency of occurrence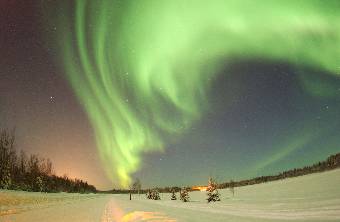
The aurora is a common occurrence in
the ring-shaped zone. It is occasionally seen in temperate
latitudes, when a strong magnetic storm temporarily expands the
auroral oval. Large magnetic storms are most common during the peak
of the 11-year sunspot cycle, or during the 3 years after that peak.
However, within the auroral zone the likelihood of an aurora
occurring depends mostly on the slant of interplanetary magnetic
field lines (further below), being greater with southward slants.
Geomagnetic storms that ignite auroras actually happen more often
during the months around the equinoxes -- that is, early Autumn and
Spring. It's a bit puzzling because polar activity normally does not
depend on the Earth's seasons. Why should geomagnetic storms?
"We've known about this seasonal effect for more than 100 years,"
says Dennis Gallagher, a space physicist at the NASA Marshall Space
Flight Center. "Some aspects of it are understood, but not all."
Spring and Fall are good times to spot Northern Lights, and
scientists would like to know why. "At such times the two fields
(Earth's and the IMF) link up," says Christopher Russell, a
Professor of Geophysics and Space Physics at UCLA. "You can then
follow a magnetic field line from Earth directly into the solar
wind." South-pointing Bz's open a door through which energy from the
solar wind can reach Earth's inner magnetosphere.
In the early 1970's Russell and colleague R. L. McPherron recognized
a connection between Bz and Earth's changing seasons: The average
size of Bz is greatest each year in early Spring and Autumn. What
remains is a puzzle that space scientists are still trying to solve.
"This is an area of active research," notes Tony Lui, a space
physicist at the Johns Hopkins University Applied Physics Lab. "We
don't have all the answers yet, because it's a complicated problem."
ref: NASA
return to top
The solar wind and magnetosphere
The Earth is constantly immersed in
the solar wind, a rarefied flow of hot [1] plasma (gas of free
electrons and positive ions) emitted by the sun in all directions, a
result of the million-degree heat of the sun's outermost layer, the
solar corona. The solar wind usually reaches Earth with a velocity
around 400 km/s, density around 5 ions/cc and magnetic field
intensity around 2–5 nT (nanoteslas; the Earth's surface field is
typically 30,000–50,000 nT). These are typical values. During
magnetic storms, in particular, flows can be several times faster;
the interplanetary magnetic field (IMF) may also be much stronger.
The IMF originates on the sun, related to the field of sunspots, and
its field lines (lines of force) are dragged out by the solar wind.
That alone would tend to line them up in the sun-earth direction,
but the rotation of the Sun skews them (at Earth) by about 45
degrees, so that field lines passing Earth may actually start near
the western edge ("limb") of the visible sun.
The Earth's "magnetosphere" is the space region dominated by its
magnetic field. It forms an obstacle in the path of the solar wind,
causing it to be diverted around it, at a distance of about 70,000
km (before it reaches that boundary, typically 12,000–15,000 km
upstream, a bow shock forms). The width of the magnetospheric
obstacle, abreast of Earth is typically 190,000 km, and on the night
side a long "magnetotail" of stretched field lines extends to great
distances.
return to top
The origin of the aurora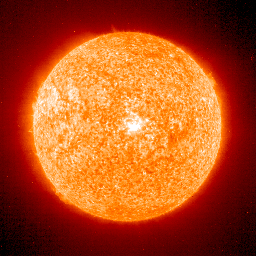
The ultimate energy source of the
aurora is undoubtedly the solar wind flowing past the Earth.
Both the magnetosphere and the solar wind consist of plasma, which
can conduct electricity. It is well known (since Faraday's work
around 1830) that if two electric conductors are immersed in a
magnetic field and one moves relative to the other, while a closed
electric circuit exists which threads both conductors, then an
electric current will (usually) arise in that circuit. Electric
generators of dynamos make use of this process ("the dynamo
effect"), but the conductors can also be plasmas or other fluids.
In particular the solar wind and the magnetosphere are two
electrically conducting fluids with such relative motion, and should
be able (in principle) to generate electric currents by "dynamo
action," in the process also extracting energy from the flow of the
solar wind. The process is hampered by the fact plasmas conduct
easily along magnetic field lines but not so perpendicular to them.
It is therefore important that a temporary interconnection be
established between the field lines of the solar wind and those of
the magnetosphere, by a process known as magnetic merging or
reconnection. It happens most easily with a southward slant of
interplanetary field lines, because then field lies north of Earth
approximately match the direction of field lines near the north
magnetic pole (namely, into the earth), and similarly near the
southern pole. Indeed, active auroras (and related "sub-storms") are
much more likely at such times.
Electric currents originating in such fashion apparently give
auroral electrons their energy. The magnetospheric plasma has an
abundance of electrons: some are magnetically trapped, some reside
in the magnetotail, and some exists in the upwards extension of the
ionosphere, which may extend (with diminishing density) some 25,000
km around the Earth.
Bright auroras are generally associated with Birkeland currents (Schield
et al., 1969; Zmuda and Armstrong, 1973) which flow down into the
ionosphere on one side of the pole and out on the other. In between,
some of the current connects directly through the ionospheric E
layer (125 km), the rest ("region 2") detours, leaving again through
field lines closer to the equator and closing through the "partial
ring current" carried by magnetically trapped plasma. The ionosphere
is an ohmic conductor, so such currents require a driving voltage,
which some dynamo mechanism can supply. Electric field probes in
orbit above the polar cap suggest voltages of the order of 40,000
volts, rising up to more than 200,000 volts during intense magnetic
storms.
Ionospheric resistance has a complex nature, and leads to a
secondary "Hall current" flow. By a strange twist of physics, the
magnetic disturbance on the ground due to the main current almost
cancels out, so most of the observed effect of auroras is due to a
secondary current, the auroral electrojet. An "auroral electrojet"
(AE) index (measured in nanotesla) is regularly derived from ground
data, and serves as a general measure of auroral activity.
However, ohmic resistance is not the only obstacle to current flow
in this circuit. The convergence of magnetic field lines near Earth
creates a "mirror effect" which turns back most of the down-flowing
electrons (where currents flow upwards), inhibiting current-carrying
capacity. To overcome this, part of the available voltage appears
along the field line ("parallel to the field"), helping electrons
overcome that obstacle by widening the bundle of trajectories
reaching Earth; a similar "parallel voltage" is used in "tandem
mirror" plasma containment devices. A feature of such voltage is
that it is concentrated near Earth (potential proportional to field
intensity; Persson, 1963) and indeed, as deduced by Evans (1974) and
confirmed by satellites, most auroral acceleration occurs below
10,000 km. Another indicator of "parallel electric fields" along
field lines are beams of upwards flowing O+ ions observed on auroral
field lines.
While this mechanism is probably the main source of the familiar
auroral arcs, formations conspicuous from the ground, more energy
might go to other, less prominent types of aurora, e.g. the diffuse
aurora (below) and the low-energy electrons precipitated in magnetic
storms (also below).
Some O+ ions ("conics") also seem accelerated in different ways by
plasma processes associated with the aurora. These ions are
accelerated by plasma waves, in directions mainly perpendicular to
the field lines. They therefore start at their own "mirror points"
and can travel only upwards. As they do so, the "mirror effect"
transforms their directions of motion, from perpendicular to the
line to lying on a cone around it, which gradually narrows down.)
In addition, the aurora and associated currents produce a strong
radio emission around 150 kHz (AKR or "auroral kilometric
radiation") discovered in 1972. Ionospheric absorption makes AKR
observable from space only.
These "parallel voltages" accelerate electrons to auroral energies
and seem to be a major source of aurora. Other mechanisms have also
been proposed, in particular, Alfvén waves, wave modes involving the
magnetic field first noted by Hannes Alfvén (1942), which have been
observed in the lab and in space. The question is however whether
this might just be a different way of looking at the above process,
because this approach does not point out a different energy source,
and many plasma bulk phenomena can also be described in terms of
Alfvén waves.
Other processes are also involved in the aurora, and much remains to
be learned. Auroral electrons created by large magnetic storms often
seem to have energies below 1 keV, and are stopped higher up, near
200 km. Such low energies excite mainly the red line of oxygen, so
that often such auroras are red. On the other hand, positive ions
also reach the ionosphere at such time, with energies of 20-30 keV,
suggesting they might be an "overflow" along magnetic field lines of
the copious "ring current" ions accelerated at such times, by
processes different from the ones described above.
return to top
Sources and types of aurora
Again, our
understanding is very incomplete. A rough guess may
point out three main sources:
Dynamo action with the solar wind flowing past
Earth, possibly producing quiet auroral arcs
("directly driven" process). The circuit of the
accelerating currents and their connection to the
solar wind are uncertain.
Dynamo action involving plasma squeezed earthward by
sudden convulsions of the magnetotail ("magnetic
substorms"). Sub-storms tend to occur after
prolonged spells (hours) during which the
interplanetary magnetic field has an appreciable
southward component, leading to a high rate of
interconnection between its field lines and those of
Earth. As a result the solar wind moves magnetic
flux (tubes of magnetic field lines, moving together
with their resident plasma) from the day side of
Earth to the magnetotail, Widening the obstacle it
presents to the solar wind flow, and causing it to
be squeezed harder. Ultimately the tail plasma is
torn ("magnetic reconnection") --some blobs ("plasmoids")
are squeezed tailwards and are carried away with the
solar wind, others are squeezed earthwards where
their motion feeds large outbursts of aurora, mainly
around midnight ("unloading process").
Magnetic storms have similar effects, but with
greater vigor. The big difference is the addition of
many particles to the plasma trapped around Earth,
enhancing the "ring current" which it carries. The
resulting modification of the Earth's field allows
aurora to be visible at middle latitudes, on field
lines much closer to the equator.
Satellite images of the aurora from above show a
"ring of fire" along the auroral oval (see above),
often widest at midnight. That is the "diffuse
aurora," not distinct enough to be seen by the eye.
It does not seem to be associated with acceleration
by electric currents (although currents and their
arcs may be embedded in it) but to be due to
electrons leaking out of the magnetotail.
Any magnetic trapping is leaky--there always exists
a bundle of directions ("loss cone") around the
guiding magnetic field lines where particles are not
trapped but escape. In the radiation belts of Earth,
once particles on such trajectories are gone, new
ones only replace them very slowly, leaving such
directions nearly "empty." In the magnetotail,
however, particle trajectories seem to be constantly
reshuffled, probably when the particles cross the
very weak field near the equator. As a result the
flow of electrons in all directions is nearly the
same ("isotropic"), and that assures a steady supply
of leaking electrons.
The energizing of such electrons comes from
magnetotail processes. The leakage of negative
electrons does not leave the tail positively
charged, because each leaked the electron lost to
the atmosphere is quickly replaced by a low energy
electron drawn upwards from the ionosphere. Such
replacement of "hot" electrons by "cold" ones is in
complete accord with the 2nd law of thermodynamics.
Other types of aurora have been observed from space,
e.g. "poleward arcs" stretching sunward across the
polar cap, the related "theta aurora," and "dayside
arcs" near noon. These are relatively infrequent and
poorly understood. Space does not allow discussion
of other effects such as flickering aurora, "black
aurora" and subvisual red arcs. In addition to all
these, a weak glow (often deep red) has been
observed around the two polar cusps, the "funnels"
of field lines separating the ones that close on the
day side of Earth from lines swept into the tail.
The cusps allow a small amount of solar wind to
reach the top of the atmosphere, producing an
auroral glow.
return to top
Aurorae on other planets
Both Jupiter and
Saturn have magnetic fields much stronger than
Earth's (Uranus, Neptune and Mercury are also
magnetic), and both have large radiation belts.
Aurora has been observed on both, most clearly with
the Hubble telescope.
These auroras seem, like Earth's, to be powered by
the solar wind. In addition, however, Jupiter's
moons, especially Io, are also powerful sources of
auroras. These arise from electric currents along
field lines ("field aligned currents"), generated by
a dynamo mechanism due to relative motion between
the rotating planet and the moving moon. Io, which
has active volcanism and an ionosphere, is a
particularly strong source, and its currents also
generate radio emissions, studied since 1955.
An aurora has recently been detected on Mars, even
though it was thought that the lack of a strong
magnetic field would not make one possible.
return to top
Legal Notes:
This article is licensed under the
GNU Free Documentation License. It uses material
from the Wikipedia article:
http://en.wikipedia.org/wiki/Aurora_boreal
Images used are either in the public domain,
licensed under the GFDL, or permitted for use by
their originator.
|